Ultrafast Quasiparticle Dynamics of Strongly Correlated Electronic Systems
-
Background -
The power of ultrafast optical spectroscopy (UOS) lies in its ability
to temporally resolve phenomena at the fundamental timescales of atomic
and electronic motion, and unravelling the competing degrees of
freedom. In condensed matter physics, sub-picosecond temporal
resolution combined with spectral selectivity enables studies of
electronic, spin and lattice dynamics, and more importantly, the
coupling between these degrees of freedom. In recent years, femtosecond
time-resolved spectroscopy has been shown to present an excellent
experimental alternative for studying temperature-dependent changes of
the low-lying electronic structure of superconductors and other
strongly correlated electron systems including charge density waves,
manganites, or heavy fermions. In these experiments, a femtosecond
laser pump pulse excites quasiparticles. These high-energy
quasiparticles rapidly thermalize (within 10s of femtoseconds) via
electron-electron collisions, reaching states near the Fermi energy.
The subsequent relaxation dynamics are strongly affected by the
low-energy electronic structure in these materials. The dynamics are
extracted either by time-resolved measurements of the photoinduced
changes in reflectivity (ΔR/R) or transmission (ΔT/T)
at optical frequencies, or by directly measuring conductivity dynamics,
with the probe wavelength in the terahertz (far-infrared) range.
-
Technique-
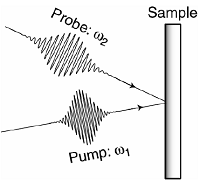 The figure
on the right depicts, in general terms, an UOS experiment. By changing
the relative delay of the pump and probe pulses it is possible to
temporally measure the subsequent relaxation dynamics. In UOS the pump
and the probe pulses need to be derived from the same pulse, typically
at 1.5 eV, to insure ultrafast time resolution but nonlinear optics can
be used to produce pulses with very different frequencies, including
subpicosecond, broadband terahertz (THz) pulses with frequencies from
~0.1-10 THz. Coherent detection offers the ability to measure both the
amplitude and phase of the THz pulse after passing through a sample,
providing direct access to the complex permittivity and conductivity of
the sample over the measured frequency range without Kramers-Kronig
analysis. When combined with a synchronized optical pump pulse,
optical-pump THz-probe spectroscopy (OPTP), in which the transmitted
THz pulse is detected at a certain time delay after optical excitation
of the sample provides a powerful variant of UOS, enabling optically
induced changes in the complex conductivity, σ real(ω) + i σ imag(ω) to be measured with sub-picosecond time resolution. For superconductors, σ imag(ω)
reveals a 1/ω dependence whose amplitude is proportional to the
superconducting condensate at a given time while σ real(ω) is proportional to the quasiparticle population.
-
Equipment -We
now have a (a) 80 MHz Ti:sapphire oscillator (KM Labs Griffin), (b) 250
kHz amplifier (Coherent RegA) with visible and mid-infrared OPAs, and
(c) 1 kHz/3.5 mJ
amplified laser system (Coherent Legend-Elite). We also have optical
cryostats from Janis (ST-500) and Cryo Industries (XE-102) that go
down to 4
kelvin. We hope to purchase a 7 tesla superconducting magnet in
the near future.
Our all-optical pump-probe system looks like this.
-
Strongly Correlated Electronic Systems (SCES) - We plan to study the ultrafast quasiparticle dynamics of SCES such as high-Tc
cuprate superconductors and other unconventional superconductors;
multiferroic single crystals, thin films and hetrostructures; and heavy
fermions, using samples from collaborators overseas as well as from
within the Department (PAP). From these studies we hope to determine
the temperature dependence of the charge gap that opens up below a
transition temperature, and how these "hot" electrons couple to the
different degress of freedom in the material, such as phonons. We also
hope to collaborate with colleagues from outside the Department, to
study nanocomposites, chemical and biological systems.
|
|